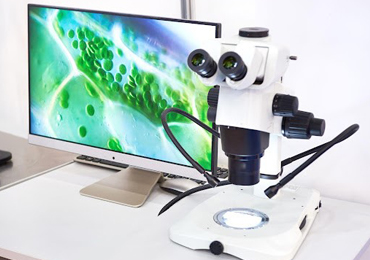
Stereo microscopes have been in use for a little over a century, but it’s not uncommon for those who routinely use them not to know how they differ from other models. Why are stereo microscopes so special?
With two optical paths and eyepieces, stereo microscopes provide a three-dimensional view of the specimen. Like our eyes, the optical paths converge onto the sample, coming to the focus point from different angles, providing a sense of depth that makes stereo microscopes so beneficial for dissecting applications. They also have a long working distance which allows easy access to the sample with tools. For this reason, stereo microscopes are also referred to as dissecting microscopes.
Let’s take a more in-depth look at stereo microscopes, how they work, and in which applications they should be used.
Parts of a Stereo Microscope
Every component of the stereo microscope is important to its unique function. Its parts may vary depending on the configuration and use of the microscope, but an average classroom stereo microscope will have the following components:
- Eyepieces: The microscope’s eyepieces are also called ocular lenses and are the part the viewer looks through to examine the specimen. The eyepieces are normally set at 10x magnification, but some models can reach up to 75x magnification.
- Diopter setting: This microscope component helps prevent eye strain by making up for the differences between the image seen through the left and right eyes.
- Objective lens: Each eyepiece of the stereo microscope is connected to its own objective lens. The microscope’s magnification level can be determined by a single fixed objective, a rotating multiple lens turret, or a zoom.
- Stereo head: This component holds the two eyepieces. In one optical design (Greenough), the objective lenses and stereo head are housed together in a single body. In the second major optical design (Common main objective or Galilean), the head mounts to the top of the optical zoom body.
- Focus knob: Stereo microscopes are commonly equipped with at least one focus knob. This knob is used to move the stereo head up and down to sharpen the image of the sample.
- Top lighting: Many microscopes only have one source of lighting, but the stereo microscope has a top and bottom light. The top light illuminates the object from above. Top lighting can be built into the microscope stand, or can be delivered by an external source either attached to the bottom of the microscope (like a ring light) or by fiber-optic bundles that direct light where the user needs it (refer to the feature image for this article).
- Bottom lighting: The bottom light of the microscope shines light through the stage and improves the visibility of translucent objects.
- Stage plate: The stage plate is the spot where the specimen is placed. It is directly underneath the objective lens. The plate is often reversible with one black side and one white side to contrast the specimen.
- Stage clips: These clips are designed to hold any slides in place on the stage.
How Does a Stereo Microscope Work?
The stereo microscope is an optical light microscope that uses the light reflected off the specimen to create a clear image of the objects’ individual details. It magnifies things at low power and is commonly used with solid or thick samples.
As the light reflects off the object, the viewer’s left and right eyes see different angles of the same sample, creating a three-dimensional image that is more informative for viewing detail and surface structure than a microscope with a single objective.
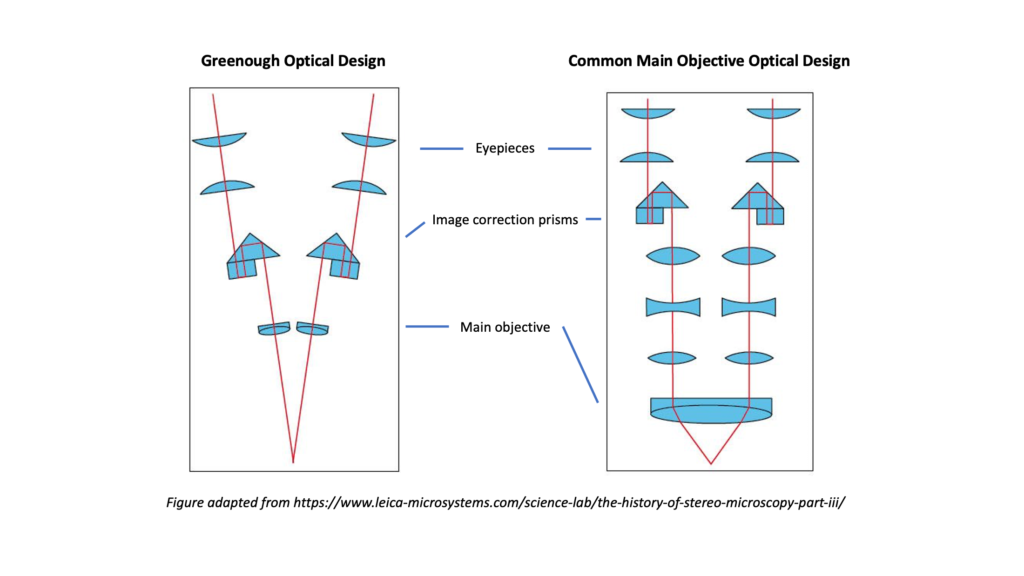
When Should a Stereo Microscope Be Used?
Because a stereo microscope allows the examination of three-dimensional specimens with its two separate optical paths and long working distance, it can be used in a variety of applications. For example, biologists and students can use these microscopes to perform dissections, while botanists use them to examine plants. They can also aid in repairing circuit boards and watches, cleaning and analyzing fossils, and dermatological examinations.
With additional microscope accessories, the stereo microscope can be configured to match a variety of applications. Boom stands, flex arms, track stands, and table mounts can improve the microscope’s ease of use, and microscope illuminators can enhance the specimen’s image and provide needed contrast.
Looking for Stereo Microscopes? Turn to ACCU-SCOPE
If you’re looking for high-quality stereo microscopes for your research, medical, or instructional purposes, trust ACCU-SCOPE to meet your needs. We have some of the best microscopes in the industry! If you need higher magnifications, we also offer compound microscopes. Contact us today to receive a quote.